Nutrition Solutions to Counter Health Impact of Air Pollution: Scientific Evidence of Marine Omega-3 Fatty Acids and Vitamins Alleviating Some Harmful Effects of PM2.5
Weiguo Zhang, Manfred Eggersdorfer, Norman Salem Jr, Jiong Jack Chen, Szabolcs Peter and Li-Qiang Qin
Affiliation
- 1DSM Nutrition Products, Human Nutrition and Health, Beijing, China
- 2DSM Nutrition Products, Human Nutrition and Health, Kaiseraugst, Switzerland
- 3DSM Nutrition Products, Nutritional Lipids, Columbia, MA, USA
- 4DSM Nutrition Products, China Innovation Center, Shanghai, China
- 5School of Public Health, Soochow University, Suzhou, China
Corresponding Author
Weiguo Zhang, DSM Nutrition Products, Human Nutrition and Health, Beijing, China. E-mail:weiguozha@yahoo.com
Citation
Zhang, W. et al. Nutrition Solutions to Counter Health Impact of Air Pollution: Scientific Evidence of Marine Omega-3 Fatty Acids and Vitamins Minimizing Some Harms of PM2.5. (2015) J Food Nutr Sci 2(1): 58-63.
Copy rights
© 2015 Zhang, W. This is an Open access article distributed under the terms of Creative Commons Attribution 4.0 International License.
Keywords
Air pollution/pollutants; Fine particular matter (PM2.5); Vitamins Docosahexaenoic and eicosapentaenoic acids (DHA and EPA); Heart rate variability (HRV); Oxidative stress
Abstract
Inhaling polluted air, especially air containing fine particulate matter (i.e. PM2.5) constitutes an environmental risk that has proven impact on the quality and duration of human life. The objective of this article is to highlight human clinical investigations in which vitamins and marine-derived long-chain polyunsaturated fatty acids (i.e. fish oil or docosahexaenoic and eicosapentaenoic acids) were administrated to minimize certain detrimental responses to PM2.5 exposure. The results from both randomized and cohort studies in last decade demonstrated that PM2.5 exposure induced unfavorable physiological and biochemical responses (i.e. heart rate variability reduction and oxidative stress) in human body. Supplementation of fish oil, some B vitamins, vitamin E and C abrogated these responses. A large number of the world’s population are now exposed to air pollution daily, which will largely remain for many years. These studies hold promise for nutrition to play a role in ameliorating some detrimental responses to PM2.5. Future investigations are warranted to determine whether long-term administration of these nutrients improves PM2.5-related clinical endpoints e.g. cardiovascular and respiratory outcomes.
Introduction
Air pollution is one of the most important environmental issues in today's world. To address its importance, the World Health Organization (WHO) published the Global Update of Air Quality Guidelines (AQG) in 2005, in which the WHO has seriously emphasized that "clean air is considered to be a basic requirement of human health and well-being" (WHO 2005).
Major contaminants in the atmosphere include particulate matter, ozone, nitrogen dioxide and sulfur dioxide, which are mainly emitted when fossil fuels (especially coal and petroleum) and biomass are combusted. Among the major pollutants, particulate matter with aerodynamic diameter smaller than 2.5 micrometers is referred to as PM2.5. PM2.5 consists of a complex mixture of solid and liquid components, with organic and inorganic substances including sulfate, nitrates, ammonia, sodium chloride, carbon, mineral dust and water, which can travel deep into the lungs when inhaled. While the ambient PM2.5 concentration is reported hourly and daily in some countries, the use of an annual mean is preferred for evaluating its long-term impact on human health. According to the AQG, the recommended PM2.5 concentration, selected to minimize likely health effects based on existing literature, is an annual mean of10 μg/m3 and a 24 hours mean of 25 μg/m3. The WHO also sets an annual mean of 35 μg/m3 as an interim target-1 (IT-1).
In this two-part literature review, we first describe an overview of current understanding about the harmful effects of PM2.5 and then summarize data from human studies on nutritional solutions as intervention for the detrimental responses to PM2.5 exposure. To identify the appropriate studies for inclusion for the second part, only scientific publications in peer-reviewed journals were considered, and the PubMed database was used. Specific nutritional interventions included vitamins and marine-derived long-chain polyunsaturated fatty acids (LCPUFA, i.e. fish oil or omega-3 fatty acids). Studies targeting populations composed exclusively of cigarette smokers were excluded, as cigarette smoke largely represents a self-induced PM2.5 exposure in addition to air pollution and is better managed differently (e.g. by lifestyle modification). Studies without human subjects were cited only when mechanisms were introduced.
Overview of Health Harms of PM2.5
PM2.5 is a Human Health Threat
According to the 2005 WHO Guidelines, exposure to PM2.5 at the 35 μg/m3 level (i.e. IT-1) is associated with an ap-proximately 15% increase in mortality risk, relative to the AQG of 10 μg/m3. A statement made by the American Heart Association in 2010, concluded that "the overall evidence from the cohort studies demonstrates on average an approximate 10% increase in all-cause mortality per 10 μg/m3 elevation in long-term average PM2.5 exposure"[1].
Carrying many toxic substances and getting to the alveoli deep within the lungs, PM2.5 can evoke a series of responses locally and systemically when inhaled. At the cellular and molecular level, exposure to PM2.5 causes an increased inflammatory response and a decreased anti-oxidative capability which would normally counteract reactive oxygen species (ROS), leading to oxidative stress[2,3]. Some investigations also showed that more antioxidants in circulation system are consumed in response to air pollution[3-6]. Decreased antioxidant capability or increased oxidative stress is repeatedly implicated as an important (if not the sole) mechanism[1,7,2,8] in translating toxicity of PM2.5 into disease pathogenesis.
An example of PM2.5-induced cardiovascular damage is endothelial dysfunction and accelerated development of atherosclerosis[9,10]. In a rodent model, larger arterial atherosclerotic plaque was demonstrated by histopathology after apoE deficient mice exposed to PM2.5 at a concentration of 85 μg/m3 for 6 hours/day, 5 days/week for 6 months versus the same animals that had filtered clean air. In the recent Multi-Ethnic Study of Atherosclerosis (MESA) with 5,362 human subjects over age 60, the intima-medial thickness (IMT) of carotid artery was examined by ultrasound as a surrogate marker for arterial hypertrophy and lumen narrowness as well as atherosclerotic development[11]. The results from the MESA population showed that the average annual progression of carotid IMT was 14 μm. An increase of 2.5 μg/m3 in residential PM2.5 level was associated with an additional 5 μm increase in IMT progression annually. This concentric arterial hypertrophy narrows arterial lumen, therefore causing hemodynamic disorders (e.g. blood flow turbulence and blood flow reduction in the distal end of the artery) and augmenting the risk of vascular events (e.g. thrombosis, vessel spasm and ischemia). An example of PM2.5-induced respiratory damage is the increased risk of lung cancer development. A recent meta-analysis from ESCAPE (European Study of Cohorts for Air Pollution Effects), showed a statistically significant association between risk for lung cancer and PM2.5 concentration with hazard ratio (HR) of 1.18 (95% CI 0.96 - 1.46) per 5 μg/m3 increase (for PM10, the HR was 1.22 with 95% CI 1.03 - 1.45 per 10 μg/m3). For lung adenocarcinomas, with the same increments of PM10 and PM2.5, HRs were 1.51 (1.10 - 2.08) and 1.55 (1.05 - 2.29), respectively
PM2.5 Pollution is a Worldwide Issue
While the best measure of PM2.5 is from instruments in-situ, satellite data was found to be well correlated with surface measurements, allowing for a global estimation of PM2.5[12].
During 2001-2006, a global population-weighted geometric mean PM2.5 concentration was estimated to be 20 μg/m3, which is two-fold higher than the AQG of 10 μg/m3. In the estimation, the WHO PM2.5 interim target-1 (i.e. 35 μg/m3 annual average) was exceeded over central and eastern Asia for 38% and for 50% of the respective populations[12]. In some particular regions, annual mean PM2.5 concentrations exceeded 80 μg/m3. Overall, it was estimated that 80% of the world populations lived in regions that exceeded the AQG[12].
PM2.5 pollution is a long-term issue.
To control and reduce PM2.5 emission at its source is ideal, but very challenging to practically implement. In the United States for example, according to the Environmental Protection Agency, it took over 12 years (from 2000 to 2012) to make a modest 37% reduction in PM2.5 concentration; in large metropolitan areas, however, PM2.5 remains an important pollutant[12].
In developing countries, such as China, even the most aggressive plan for PM2.5 reduction would reduce PM2.5 by only 25% by 2017 (compared to 2012 levels in the Beijing-Tianjin and Hebei area); even if this goal is achieved by then, the annual PM2.5 concentration is projected to be 60 μg/m3, which is still 6-fold higher than the AQG recommendation.
Clearly, reduction of PM2.5 emission requires many coordinated efforts and resources including education, novel and affordable technology, legislation and law enforcement, and most importantly, time. Therefore, in the interim, large populations remain exposed to levels of PM2.5 above the WHO recommendations on a daily basis.
Nutrition on PM2.5-Associated Heart Rate Variability
Heart rate variability (HRV) or cardiac cycle length variability is a measurement of interval oscillations between consecutive heartbeats, reflecting beat-to-beat control in the heart by autonomic regulation. Generally, HRV declines with aging. In clinical settings, a reduction of HRV predicts adverse cardiovascular outcomes including ventricular arrhythmia, sudden cardiac death and myocardial infarction[13-15]. HRV is derived from an electrocardiogram (ECG) that registers each QRS complex. Between adjacent QRS complexes, so-called normal- to-normal intervals (all intervals resulting from sinus node cyclic depolarization) are calculated. The standard deviations of normal-to-normal intervals (SDNN) reflect the time-domain differences of cardiac cycles in the period of ECG recording. Other HRV indices are low frequency (LF) and high frequency (HF). Both LF and HF are derived from power spectral density (PSD) analysis and provide information about how power (variance) distributes as a function of frequency. The HF and LF are considered to predominantly reflect parasympathetic (vagal) nerve activity and sympathetic drive (with some parasympathetic influence), respectively, from the central nervous system to the heart[16,17].
The link between PM2.5 exposure and HRV abnormalities was noticed in late 1990s and confirmed repeatedly afterwards[18-22]. For example, in the elderly residing in a nursing home in Mexico City where daily indoor and outdoor PM2.5 ranged from 15-67 and 9-87 μg/m3, a 10 μg/m3 increase in PM2.5 was associated with a 5.0% reduction in HRV-HF[23]. In subjects without the allele for glutathione-S-transferase M1 (GSTM1), a 10 μg/m3 increase in PM2.5 during the 48 hours before HRV measurement was associated with as much as 34% decrease in HRV-HF (95% confidence interval from −9% to −52%)[24]. This polymorphic "null" genotype was reported in about half of the Caucasian population[25].
Fish Oil Prevents HRV Reduction Associated with PM2.5
In a double-blind clinical trial, 50 subjects over 60 years old were randomized to receive 2 g fish oil containing 52% docosahexaenoic acid (C22:6 n-3 DHA) and 25% eicosapentaenoic acid (C20:5 n-3 EPA) or 2 g soy oil for the placebo arm for a period of 6 months (1 month pre-supplementation and 5 months supplementation)[26]. During this time, the ambient PM2.5 levels varied from 5.1 to 49 μg/m3. The 24-hour average of PM2.5 concentration was 18.6 ± 8.0 μg/m3 (mean ± SD). For every 8 μg/m3 increase in PM2.5 (one standard deviation), the corresponding reduction of HRV-HF before supplementation was 54%. After 5 months of fish oil supplementation, the reduction was only 7%. In other words, a 47% reduction of HRV-HF was prevented by fish oil, compared to 31% by placebo (P = 0.11) (Figure 1). In addition, SDNN reduction was 27% before supplementation and was 0.5% after fish oil intervention (p < 0.01). The effect of placebo oil on SDNN was not statistically significant. Biochemically, there were large increases of both EPA and DHA in erythrocytes from pre-supplementation levels (394% and 140%, respectively). In contrast, soy oil supplementation resulted in a moderate increase in EPA (87%) and no statistical increase in DHA[26].
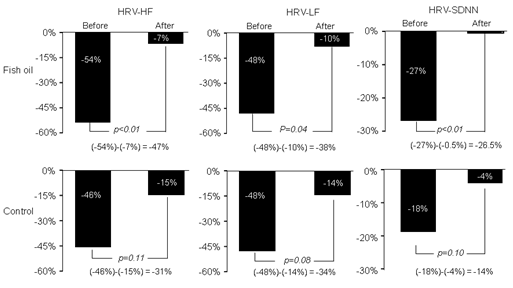
Figure 1: Indices of heart rate variability (HRV), i.e. high frequency (HF), low frequency (LF) and standard deviations of normal-to-normal intervals (SDNN) were all reduced in response to each 8 μg/m3 increase in indoor PM2.5, which was largely prevented by fish oil supplement with statistical significance.
In another randomized trial, 29 healthy, middle-aged subjects were randomly assigned to receive olive oil as placebo or 3 g fish oil (containing 65% n-3 fatty acids) daily for 4 weeks, before being placed in an experimental chamber and exposed to concentrated PM2.5 and ultrafine particles with concentration ranging from 83 to 470 μg/m3 with an average of 278 ± 19 μg/m3 [27]. After an acute exposure for 2 hours, the ratio of HF/LF of HRV was lowered and stayed low for 20 hours in subjects who had received placebo, signifying an altered balance between sympathetic and parasympathetic nerve activity. The reduction of the HF/LF ratio, however, was prevented in subjects who had received intervention with 3 g/day fish oil. Plasma assay revealed that there was more than a 5-fold increase in EPA and more than a 2-fold increase in docosapentaenoic acid (DPA) in subjects who received fish oil[27].
Dietary Methyl Nutrients May Prevent HRV Reduction Associated With PM2.5
Dietary methyl nutrients include folate, pyridoxine (vitamin B6), cyanocobalamin (B12) and methionine[28]. The effects of different intakes of methyl nutrients on PM2.5-induced HRV were investigated in the Normative Aging Study in elderly men. In all subjects, adjusted changes in SDNN, HF and LF for each 10 g/m3 of PM2.5 in the 48 hours before nutrient intake measurement were -7.1% (p = 0.03), -18.7% (p = 0.01) and -11.8% (p = 0.08), respectively. In this study, lower dietary intake of vitamin B6 (< 3.65 mg/d), B12 (< 11.1 μg/d) and methionine (< 1.88 mg/d) were accompanied by a pronounced reduction in SDNN, HF and LF for each 10 g/m3 increase in PM2.5 concentration. In contrast, higher intake of vitamin B6 (≥ 3.65 mg/d), B12 (≥ 11.1 μg/d) and methionine (≥ 1.88 mg/d) abrogated HRV reduction (Figure 2). The effects of folate intake on HRV reduction were not statistically significant. Although PM2.5 attenuated both HF and LF, the LF reduction was greater, indicating an imbalance between sympathetic and parasympathetic drive to the heart or relative activation of sympathetic nervous system. Figure 2 shows the effects of different methyl nutrients on SDNN. Similarly, the higher daily intake of these methyl nutrients prevented LF and HF from PM2.5-associated reduction. Of note, this study was not a randomized, placebo-controlled trial and the nutrient intake was estimated by dietary questionnaire, rather than precisely administered. Although the results favor increased methyl nutrient intake, these benefits are only correlations and need to be confirmed with more rigorous clinical trials.
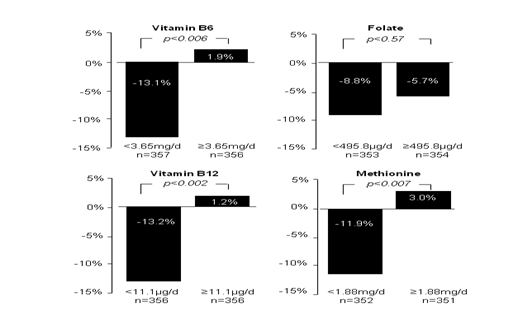
Figure 2: PM 2.5 reduced HRV-SDNN significantly in subjects who had less vitamin B6, B12 and methionine intakes. Such reductions were prevented in those who had higher methyl nutrient intakes.
Gene and environmental interactions were observed in a subset analysis. Subjects with methylene tetrahydrofolate reductase (MTHFR) 677CT and 677TT polymorphisms had lower SDNN compared with 677CC before dietary examination; each 10 g/m3 increase in PM2.5 in the 48 hours before the examination was associated with a further 8.8% decrease in SDNN in MTHFR CT and TT, which was absent in the CC genotype. In cytoplasmic serine hydroxyl methyltransferase (cSHMT) 1420CC phenotype, PM2.5 caused an 11.8% decrease in SDNN, which was absent in cSHMT 1420CT and 1420TT carriers[29]. Both MTHFR and cSHMT polymophysms were linked to cardiovascular risk as they involve in biological processes such as methyl group transfer, homosysteine metabolism and redox reactions[29-31] which were previously shown to be altered by exposure to air pollution[32,33,24]. Working as coenzymes or substrates in the methionine cycle that contributes to controlling the biological processes[28]. B vitamins and methionine may play a role in protecting this genetically vulnerable population against PM2.5-induced HRV change.
Nutrition on PM2.5–Induced Biochemical Alterations
Local and systematic inflammation and oxidative stress represent a major biochemical response to the exposure to PM2.5. To date, a large family of inflammatory biomarkers and reactive oxygen species (ROS) were unfavorably changed by PM2.5, including C-reactive protein (CRP), interleukin-6 (IL-6), IL-1β, tumor necrosis factor-α (TNF-α), superoxide dismutase (SOD), glutathione (GSH), lipid peroxidation (LPO), and glutathione S-transferase (GST). The alteration of these bioactive molecules are well known in mediating a physiological (e.g. aging) and pathological (e.g. atherosclerosis) cascade, relevant to the pathogenesis of various non-communicable diseases. While the anti-inflammatory and anti-oxidative effects of omega-3 have been recognized for a long time, some of the important mechanisms have been recently unveiled. For example, omega-3 binds to the G protein coupled receptor GPR120, alters cell membrane fatty acid composition and lipid rafts, and inhibits the activation of pro-inflammatory transcriptional factor (i.e. NF-ï