Synthesis of Nitrogen-Doped Graphene via Thermal Treatment of Graphene Oxide within Methylimidazole and its Capacitance Performance as Electric Double Layer Capacitor
Md. Monirul Islam1, Shaikh Nayeem Faisal2, Anup Kumar Roy2, Sonia Ansari2, Dean Cardillo1, Konstantin Konstantinov1 and Enamul Haque2*
Affiliation
- 1Institute for Super conducting and Electronic Materials, University of Wollongong, NSW, Australia
- 2School of Chemical & Biomolecular Engineering, The University of Sydney, NSW, Australia
Corresponding Author
Haque E, School of Chemical & Biomolecular Engineering, The University of Sydney, NSW 2006, Australia, Tel: +61416747914; E-mail: enamul.haque@sydney.edu.au
Citation
Haque, E., et al. Synthesis of Nitrogen-Doped Graphene via Thermal Treatment of Graphene Oxide within Methylimidazole and its Capacitance Performance as Electric Double Layer Capacitor. (2015) J Nanotech Mater Sci 2(1) : 1- 5.
Copy rights
© 2015 Haque. E. This is an Open access article distributed under the terms of Creative Commons Attribution 4.0 International License.
Keywords
chemical vapour deposition; Electrochemical impedance spectroscopy
Abstract
Nitrogen-doped graphene was successfully synthesised from graphene oxide (GO) and 2-methylimidazole composite via thermal treatment under argon flow at 700°C within 1h. This synthesised N-doped graphene exhibits homogeneous nitrogen doping with concentration of ~5% in three different nitrogen configuration namelypyridinic N, pyrrolic N and graphitic N. The electric double layer capacitor (EDLC) made up with this N-doped graphene showed excellent specific capacitance 274 F/g at current density of 1A/g, which was ~7 times higher than GO. This EDLC capacitor showed excellent cyclic stability up to 5000 cycles with capacity retention of ~91%.
Introduction
Two dimensional single-layer nanostructure of sp2 hybridized conjugated carbon atoms is termed as graphene[1,2]. Graphene materials have attracted an interesting research topic which usually widely used in the area to prepared high performance electrode for capacitor due to is superior electrical, mechanical and thermal properties[2-4]. Modification of graphene structure with hetero atoms can tremendously change the electronic properties with can enhance its performance in the diversity fields of applications[5] .Commonly used heteroatom namely S, N, P and B-doped graphene reported in the literature[6-8]. Among them, recently N-doped graphene has attracted attentions due to is various applications[9,10]. The doping of nitrogen (N) atom in graphene structure can enhance the electron mobility which leads to a larger capacitance because of its strong valance bond and atomic size[11]. Nitrogen doping in graphene structure easily influence the local electronic structures[12,13] which help to improves the device performance in many types of applications including fuel cells[14], electronic devices[15] and biosensors[16].
N-doped graphene can be synthesised in various pathways such as nitrogen plasma process,[17,9] arc-discharge[18], segregation growth[19], chemical vapour deposition (CVD)[20,21], thermal annealing[22,23], and hydrothermal process[24,25]. Among these process, the thermal annealing of graphene oxide with a solid source of nitrogen are attractive because they can be scaled up without any catalyst[26]. On the other hand, most of the other techniques use gaseous or liquid sources of nitrogen to synthesis nitrogen doped graphene, and the method also suffers from practical limitations due to possible contamination from the metal deposition catalyst as well as the toxicity of the precursors.
In this work we synthesis nitrogen doped graphene using a solid nitrogen precursors 2-methylimidazole via thermal treatment under argon flow. To the best of our knowledge, we are the first time synthesis N-doped graphene using 2-methyimidazole precursors. In this process graphene oxide (GO) and 2-methylimidazole composite were heated up to 700oC for 1h to get N-doped graphene which shows excellent capacitance properties as electric double layer capacitor (EDLC).
Experiment
Graphene oxide (GO) was synthesised according to the literature reported method[27]. In details, graphite flakes (Asbury Carbons, Carbon content: 99%) were put in a ceramic boat and then placed in tube furnace at 1050oC for 15second to get thermally expanded graphite. Then 2gm of expandable graphite and 250mL of H2SO4 (98%, Sigma Aldrich) were taken in a three neck flask and stirred for 24h at room temperature. After that, 6 gm of KMnO4 (99%, Sigma Aldrich) added slowly under continuous stirring. Then the solution mixture was kept 24h under stirring. The mixture was then taken under ice bath and 250mL deionised water and 60mL of H2O2 (30%, Sigma Aldrich) were drop wise added in the mixture under stirring. The resulting solution mixture changes the colour to light brown. Finally GO dispersion was washed 3 times with HCl solution (9H2O:1HCl vol%) at 6000rpm centrifugation process. The GO precipitation was washed 10 times with water till to get pH = 6. Then the GO was collected and dried at 80oC in vacuum oven for 12h.
Nitrogen-doped graphene was synthesis from GO-methylimidazole composite materials. For making this composite, 70mg GO and 30mg 2-methylimidazole (Sigma-Aldrich, 99%) was mixed in 50mL H2O and then it was dispersed under sonication for 1h. Then the solution mixture was heated upto 80oC for 12h under stirring till to remove the water. Then the final solids (GO-methylimidazole composite) were collected and put ceramic quartz boat. Then the ceramic boat was placed in tube furnace at 700oC under argon flow for 1h. Then the final product stored as nitrogen doped graphene.
The phase structures of synthesized graphene materials were examined by X-ray diffraction (XRD, GBC MMA, Cuk α radiation, λ = 0.154 nm). The morphology of the materials and Energy dispersive spectroscopy (EDS) mapping of element present in N-doped graphene were observed by field emission electron microscope (FE-SEM, JEOL JSM-7500FA), the surface chemical compositions were analysis by X-ray photoelectron spectroscopy (XPS, SPECS GmbH). Raman spectroscopy (Renishaw) was performed r at λ = 514.5 nm). The transmission electron microscopy (TEM) was examined by JEOL F3000 instrument.
All the electrochemical characterization of the electric double layer capacitor (EDLC) was performed by VMP3 Bio-Logic electrochemical workstation. Then cyclic volt ammograms (CVs) and galvanostatic charge-discharge were measured at scan rate of 100mV/s and current density of 1 A/g respectively. The electrochemical impedance spectroscopy (EIS) measurement was performed at room temperature where the frequency range spanned 100kHz to 0.01Hz. For making EDLC device, two gold coins (15mm in diameter) were used as electrodes and the coated electrode were separated by glass fibre separator. The separator was placed between the coated electrodes that were pressed together at high pressure and 0.5M H2SO4 was used as electrolyte in the air tight cell. The electrode was prepared by loading 90% active material and 10% PTFE (polytetrafluoroethylene) binder. 0.5mg active materials were deposited on the each gold electrode surface using a 10wt% PTFE binder. The total loading of the active materials of the cell was 1mg. Capacitance was calculated from CV by the following equation[28]. Capacitance, C = 4 × (Current / Scan rate ),where Current = (|Ia| + |Ic|)/2, Ia = anodic current and Id = cathodic current. Specific capacitance calculated by the following equation: Cs = C/m, Where m is the total weight of active material of two electrodes. The gravimetric capacitance from galvanostatic charge/discharge was calculated using the following equation[29,30].
Where Cs is specific capacitance (F/g), I is the charge/discharge current density (A/g), m is the total mass of materials deposited on the two electrodes and dV/dt was calculated from the slope of the discharge curve.
Result and Discussion
The synthesised graphene oxide GO and N-doped graphene were characterized by X-ray diffraction. It can be observed from (Figure 1), GO showed peak at 2θ = 11.6o which is very close to the reported XRD pattern of GO[31] . On the other hand, after thermal treatment of GO- methylimidazole composite, the XRD peak position shifted to 2θ = 26.3o similar to the XRD peak position of N-doped graphene[31]. That result illustrates that the dspacing of GO (d= 0.762nm) is decreasing after thermal treatment of GO-methylimidazole composite, that means a significant removal of oxygen groups was occurred during the process and GO was reduced into the N-doped graphene (d = 0.336nm)[32]. In addition, the peak of N-doped graphene at 2θ = 26.3o with broader width and weaker intensity than pristine graphene oxide (2θ = 11.6o) is due to the defect produced by the insertion of nitrogen atom and carbon atom loss destroy the graphite crystal structure that means the degree of disorder increased within the graphene sheet. The decreasing of the oxygen group can be easily understood from the XPS analysis.(Figure 2a) showed the XPS spectrum of GO which composed of two elements C and O; on the other hand after thermal treatment of GO-methylimidazole, three elements C, O and N was found (Figure 2b). C and O content was found 65.1% and 34.8% respectively in GO materials, and 88.1% C and 7.0% O was found in after thermal annealing of the GO-methylimidazole composite. The C/O ratio (= 1.86) of GO was increased much after thermal treatment of the composite and was reached to 12.56 which demonstrate the reduction of GO and the presence of N means the successful synthesis of N-doped graphene.
Table 1: Chemical composition and capacitance performance of graphene oxide (GO) and N-doped graphene
Materials | Surface chemical concentration (at%)a | Capacitanceb (F/g) | |||
---|---|---|---|---|---|
C | O | N | C/O | ||
GO | 65.13 | 34.87 | -c | 1.87 | 38 |
N-doped graphene | 88.10 | 7.01 | 4.89 | 12.56 | 274 |
aCalculated from XPS analysis refer to Figure: 2;
bCalculated from galvanostatic charge-discharge profile refer to Figure 9b;
cNot detected.
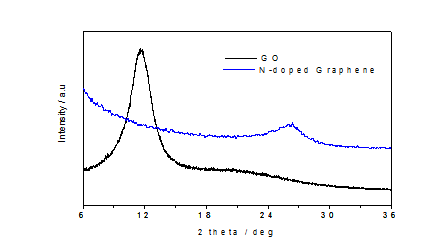
Figure 1: X-ray diffraction patterns: (a) graphene oxide (GO); (b) nitrogen-doped graphene after thermal treatment of graphene oxide and 2-methylimidazolecomposite at 700°C for 1h
The morphology of these two materials (GO and N-doped graphene) was observed by SEM. From the SEM image(Figure 3), it can be seen that the GO sheets was found more stacking of sheets, but the N-doped graphene sheets were more separated flake like a good lamellar structure, and rich wrinkles structures on the surface for the usual N-doped graphene[31]. The TEM images of GO and N-doped graphene exhibit a sheet like structure. Go exhibit more likely agglomerated with multiple sheets than that of N-doped graphene. It can be clearly found from its corresponding selected area diffraction pattern (SAED). Its SAED pattern of GO (Figure 4a) shows concentric rings masked with bright many spots demonstrating it to be of polycrystalline nature with multiple layers of graphene sheet. On the contrary, SAED pattern of N-doped graphene (Figure 4b) clearly shows the nature of graphene sheets to be crystalline with six fold symmetry illustrated the single layer graphene structure.
The bonding configurations of nitrogen atom within the carbon lattice in the structural carbon materials are mainly three types[33] quaternary N (or graphitic N), pyridinic N, and pyrrolic N (Figure 5). When the N atoms that substitute C atoms in the hexagonal rings are termed as quaternary nitrogen. Pyridinic nitrogen refers to the nitrogen that bond with two C atoms at the edge of the hexagonal ring that contribute one p electron to the π system. Pyrrolic nitrogen bonds into the five-membered ring like pyrrole that contribute two p electron into the π system[33]. The types of doped nitrogen in our synthesised N-doped graphene was observed from the deconvulated N1s region of XPS spectrum(Figure 6). N1s spectrum showed three individual peaks at binding energy at 398.3, 400.1 and 401.6 eV which is attributed to pyridinicN, pyrrolic N and quaternary N[33].This result illustrates the doping of nitrogen atom in the synthesised N-doped graphene structure. The homogeneous doping of nitrogen was confirmed by electron dispersive elemental mapping (Figure 7).GO, and the N-doped graphene were characterized by Raman spectroscopy. As shown in (Figure 8), the G peaks of the GO and N-doped graphene occurred at 1588 and 1582 cm-1. The G peaks shifted to lower energy from GO to N-doped graphene, indicating that a conjugated sp2 structure was restored during thermal treatment[34]. The ID/IG ratios of GO and N-doped graphene were 0.89 and 1.04 respectively, which were used to calculate the defects or in-plane crystallite size after thermal treatment, calculated according to the Tuinstra-Koenig (TK) relation:
La = (2.4 x 10-10) λ4 (ID/IG)–1
Where La is the crystallite sizes, λ is the Raman excitation wavelength (514 nm). The crystallite sizes of N-doped graphene (La = 16.1) was smaller than that of GO (La = 18.8) indicating that the crystallite sizes decreased upon thermal treatment.
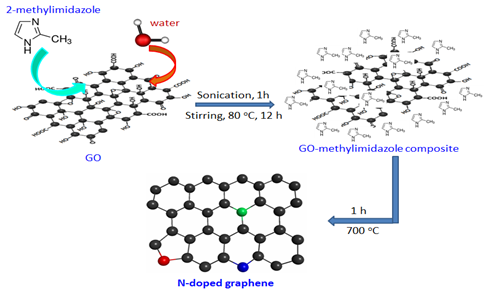
Figure 5: Synthesis process of nitrogen-doped graphene, and illustration of of types nitrogen configurations in N-doped graphene. Green, blue and red colour represents graphitic N, pyridinic N and pyrrolic N respectively.
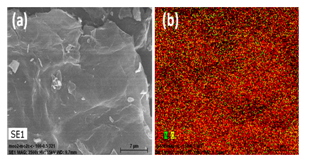
Figure 7: SEM image of N-doped graphene with its corresponding EDS elemental mapping of carbon (red), oxygen (yellow) and nitrogen (green).
Our synthesised two materials (GO and N-doped graphene) should have some differences in terms of capacitance properties as these two materials have differences in structural chemical composition. To observed the capacitance properties, electrode for double layer capacitor (EDLC) was made by GO and N-doped graphene. At first the EDLC was tested by cyclic voltammetric (CV) techniques. The (Figure 9a) showed the cyclic voltammograms (CVs) of N-doped graphene and graphene oxide (GO). Its illustrated that N-doped graphene showed much current response than GO at scan rate of 100 mV/s. The gravimetric capacitance for GO and N-doped graphene is 44 F/g and 209 F/g respectively. Capacitance of N-doped graphene is about 4 times higher than that of GO, and the rectangular shave of CV for N-doped graphene showed the ideal characteristics of double layer capacitor[35].In terms of practical application galvanostatic charge–discharge (CD) curves is the best way to measure the capacitance for actual electrode materials[36]. It also found the big difference between two materials by galvanostatic charge–discharge curves (Figure 9b). The linear regular symmetrical triangle CD curve and the longer time in CD cycle of N-doped graphene, indicates the highest capacitance for N-doped graphene than GO. The capacitance was measured from CD curves and it was 38 F/g and 274 F/g at current density of 1 A/g for GO and N-doped graphene respectively. The dramatic enhancement of capacitance for N-doped graphene is due to the doing of nitrogen in the structure of graphene; on the contrary GO was lack of nitrogen. According to the CD profile capacitance of N-doped graphene is 7 times higher than GO. The specific capacitance of this N-doped graphene is higher than N-doped graphene synthesized using literature reported precursors cyanamide[37], pyrrole[38] and urea[39]. The main reason of this big difference usually for N-doping configuration in N-doped graphene. The both quantum mechanical calculations[9,40] and experimental[41,42] investigations indicate that graphitic, pyridinic and pyrrolic N can enhance the capacitance.
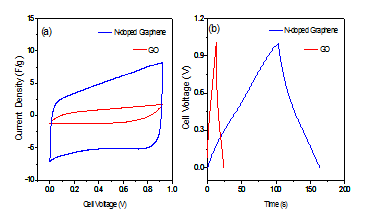
Figure 9: (a) Cyclic voltammograms (CVs) of GO and N-doped graphene EDLC device measured in 0.5 M H2SO4 at scan rate of 100 mV/s; (b) Galvanostatic charge-discharge profile of GO and N-doped graphene EDLC device measured in 0.5 M H2SO4 at current density of 1 A/g.
The cyclic stability of EDLC is very important for practical application. The capability of the electrode of EDLC device made by N-doped graphene electrodes was carried out by using galvanostatic charge discharge techniques at current density of 1 A/g(Figure 10a). It showed excellent cyclic stability up to long 5000 cycles and the capacity retention was found ~89% which is significant for an ideal capacitor (Figure 10b).
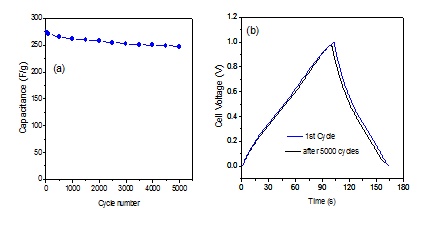
Figure 10: (a) Cycle performance of EDLC capacitor fabricated with N-doped graphene measured at 1 A/g; (b) Comparative galvanostatic charge-discharge profile between 1st cycle and after 5000th cycles.
Conclusion
In summary, nitrogen doped graphene was successfully synthesis using 2-methyimidazole as a nitrogen source for doping in graphene oxide via thermal annealing. This process was very simple and one step to synthesis N-doped graphene. The concentration of nitrogen was found ~5% with three configurations of nitrogen species. The electric double layer capacitor made up with this N-doped graphene showed high specific capacitance 274 F/g at current density of 1A/g which is ~7 times higher than GO. This capacitor showed excellent cyclic stability with capacity retention of ~91% after 5000 cycles.
Acknowledgement
Authors are grateful to the Australia research Council (ARC) for financial support and Institute for Superconducting and Electronic Materials (ISEM), University of Wollongong, for use of its infrastructure as part of in-kind support.
References
- 1. Novoselov, K.S., Geim, A.K.., Morozov, S.V., et al. Two-dimensional gas of massless Dirac fermions in grapheme. (2005) Nature 438(7065): 197–200.
- 2. Zhu, Y., Murali, S., Cai, W., et al. Graphene and graphene Oxide: Synthesis, Properties, and Applications. (2010) Adv Mater 22(35): 3906–3924.
- 3. Huang, Y., Liang, J., Chen, Y. An Overview of the Applications of Graphene-Based Materials in Supercapacitors. (2012) Small 8(12): 1805– 1834.
- 4. Zhang, Y. P., Li, D.L., Tan, X.J., et al. High quality graphene sheets from graphene oxide by hot-pressing. (2013) Carbon 54: 143–148.
- 5. Ayala, P., Arenal, R., Rummeli, M., et al. The doping of carbon nanotubes with nitrogen and their potential applications. (2010) Carbon 48(3): 575–586.
- 6. Wu, Z.S., Ren, W., Xu, L.,et al. Doped Graphene Sheets As Anode Materials with Superhigh Rate and Large Capacity for Lithium Ion Batteries.(2011) ACS Nano 5(7): 5463–5471.
- 7. Zhao, Y., Hu, C., Hu, Y., et al. A versatile, ultralight, nitrogen-doped graphene framework. (2012) Angew Chem Int Ed 51(45): 11371–11375.
- 8. Choi, C. C., Chung, M. W., Kwon, H. C., et al. B, N- and P, N-doped graphene as highly active catalysts for oxygen reduction reactions in acidic media. (2013) J Mater Chem A 1(11): 3694–3699
- 9. Jeong, H. M., Lee, J. W., Shin, W. H., et al. Nitrogen-Doped Graphene for High-Performance Ultracapacitors and the Importance of Nitrogen-Doped Sites at Basal Planes. (2011) NanoLett 11(6): 2472–2477.
- 10. Luo, G., Liu, L., Zhang, J., et al. Hole Defects and Nitrogen Doping in Graphene: Implication for Supercapacitor Applications. (2013) ACS Appl Mater Interfaces 5(21): 11184–11193
- 11. Fan, W., Xia, Y. Y., Tjiu, W. W., et al. Nitrogen-doped graphene hollow nanospheres as novel electrode materials for supercapacitor applications.(2013) J Power Sources 243: 973–981.
- 12. Biel, B., Blase, X., Triozon, F., et al. Anomalous Doping Effects on Charge Transport in Graphene Nanoribbons. (2009) Phys Rev Lett 102(9): 96803.
- 13. Huang, S. F., Terakura, K., Ozaki, T., et al. First-principles calculation of the electronic properties of graphene clusters doped with nitrogen and boron: Analysis of catalytic activity for the oxygen reduction reaction. (2009) Phys Rev B 80(235410): 1-12.
- 14. Qu, L., Liu, Y., Baek, J. B., et al. Nitrogen-Doped Graphene as Efficient Metal-Free Electrocatalyst for Oxygen Reduction in Fuel Cells.(2010) ACS Nano 4(3): 1321–1326.
- 15. Wang, X., Li, X., Zhang, L., et al. N-Doping of Graphene Through Electrothermal Reactions with Ammonia. (2009) Science 324(5928): 768–771.
- 16. Wang, Y., Shao, Y., Matson, D. W., et al. Nitrogen-Doped Graphene and Its Application in Electrochemical Biosensing. (2010) ACS Nano 4(4): 1790–1798.
- 17. Jafri,R. I., Rajalakshmi, N., Ramaprabhu, S., et al. Nitrogen doped graphene nanoplatelets as catalyst support for oxygen reduction reaction in proton exchange membrane fuel cell. (2010) J Mater Chem 20(34): 7114–7117.
- 18. Li, N., Wang, Z., Zhao, K., et al. Large scale synthesis of N-doped multi-layered graphene sheets by simple arc-discharge method. (2010) Carbon 48(1): 255–259.
- 19. Zhang, C., Fu, L., Liu, N., et al. Synthesis of Nitrogen-Doped Graphene Using Embedded Carbon and Nitrogen Sources. (2011) Adv. Mater 23(8): 1020–1024.
- 20. Wei, D., Liu, Y., Wang, Y., et al. Synthesis of N-Doped Graphene by Chemical Vapor Deposition and Its Electrical Properties. (2009) NanoLett 9(5): 1752–1758.
- 21. Jin, Z., Yao, J., Kittrell. C., et al. Tour, Large-Scale Growth and Characterizations of Nitrogen-Doped Monolayer Graphene Sheets. (2011) ACS Nano 5(5): 4112–4117.
- 22. Sheng, Z. H., Shao, L., Chen, J., et al. Catalyst-Free Synthesis of Nitrogen-Doped Graphene via Thermal Annealing Graphite Oxide with Melamine and Its Excellent Electrocatalysis.(2011) ACS Nano 5(6): 4350–4358.
- 23. Wang, X., Li, X., Zhang, L., et al. N-Doping of Graphene Through Electrothermal Reactions with Ammonia (2009) Science 324(5928): 768–771.
- 24. Wang, G., Jia, L. T., Zhu, Y., et al. Sun,Novel preparation of nitrogen-doped graphene in various forms with aqueous ammonia under mild conditions. (2012) RSC Adv 2: 11249–11252.
- 25. Chen, P., Yang, J. J., Li, S., et al. Hydrothermal synthesis of macroscopic nitrogen-doped graphene hydrogels for ultrafast supercapacitor. (2013) Nano Energy 2(2): 249–256.
- 26. Lin, Z., Waller, G., Liu, Y., et al. Facile Synthesis of Nitrogen-Doped Graphene via Pyrolysis of Graphene Oxide and Urea, and its Electrocatalytic Activity toward the Oxygen-Reduction Reaction. (2012) Adv Energy Mater 2(7): 884-888.
- 27. Pourazadi, E., Haque, E., Zhang, W., et al. Synergistically enhanced electrochemical (ORR) activity of graphene oxide using boronic acid as an interlayer spacer. (2013) Chem Commun 49(94): 11068-11070.
- 28. Antiohos, D., Folkes, D., Sherrell, P., et al. Compositional effects of PEDOT-PSS/single walled carbon nanotube films on supercapacitor device performance. (2011) J Mater Chem 21: 15987–15994.
- 29. Zhu, Y., Murali, S., Stoller, M. D., et al. Carbon-Based Supercapacitors Produced by Activation of Graphene. (2011) Science 332(6037): 1537-1541.
- 30. Islam, M. M., Chidembo, A. T., Aboutalebi, S. H., et al. Liquid crystalline graphene oxide/PEDOT:PSS self-assembled 3D architecture for binder-free supercapacitor electrodes. (2014) Front. Energy Res 2(31): 1-11.
- 31. Li, D., Yu, C., Wang, M., et al. Synthesis of nitrogen doped graphene from graphene oxide within an ammonia flame for high performance supercapacitors. (2014) RSC Adv 4: 55394–55399.
- 32. Du, X. S., Zhou, C. F., Liu, H. Y., et al. Facile chemical synthesis of nitrogen-doped graphene sheets and their electrochemical capacitance. (2013) J Power Sources 241: 460–466.
- 33. Wang, T., Maiyalagan, T., Wang, X. Review on recent progress in nitrogen-doped graphene: Synthesis, characterization, and its potential applications. (2012) ACS Catal 2(5): 781-794.
- 34. Parvez, K., Yang, S., Hernandez, Y., et al. Nitrogen-doped graphene and its iron-based composite as efficient electrocatalysts for oxygen reduction reaction. (2012) ACS Nano 6(11): 9541-9550.
- 35. Wang, T., Wang, L., Wu, D., et al. Hydrothermal synthesis of nitrogen-doped graphene hydrogels using amino acids with different acidities as doping agents. (2014) J Mater Chem A 2(22): 8352–8361.
- 36. Zhao, L., Fan, L. Z., Zhou, M. Q., et al. Nitrogen-Containing Hydrothermal Carbons with Superior Performance in Supercapacitors. (2010) Adv Mater 22(45): 5202– 5206.
- 37. Wen, Z., Wang, X., Mao, S., et al. Crumpled nitrogen-doped graphene nanosheets with ultrahigh pore volume for high-performance super capacitor. (2012) Adv Mater 24(41): 5610-5616.
- 38. You, B., Wang, L., Yao, L., et al. Three dimensional N-doped grapheme-CNT networks for supercapacitor. (2013) Chem Commun 49(44): 5016-5018.
- 39. Lei, Z., Lu, L., Zhao, X. S. The electrocapacitive properties of graphene oxide reduced by urea. (2012) Energy Environ Sci 5(4): 6391-6399.
- 40. Zhang, L. L., Zhao, X., Ji, H., et al. Nitrogen doping of graphene and its effect on quantum capacitance, and a new insight on the enhanced capacitance of N-doped carbon.Energy.(2012) Environ. Sci 5(11): 9618-9625.
- 41. Sun, Li., Wang, L., Tian, C., et al. Nitrogen-doped graphene with high nitrogen level via a one-step hydrothermal reaction of graphene oxide with urea for superior capacitive energy storage. (2012) RSC Adv 2(10): 4498-4506.
- 42. Yang, S., Feng, X., Wang, X., et al. Graphene-based carbon nitride nanosheets as efficient metal-free electrocatalysts for oxygen reduction reaction. (2011) Angew Chem Int Ed Engl 50(23): 5339-5343.